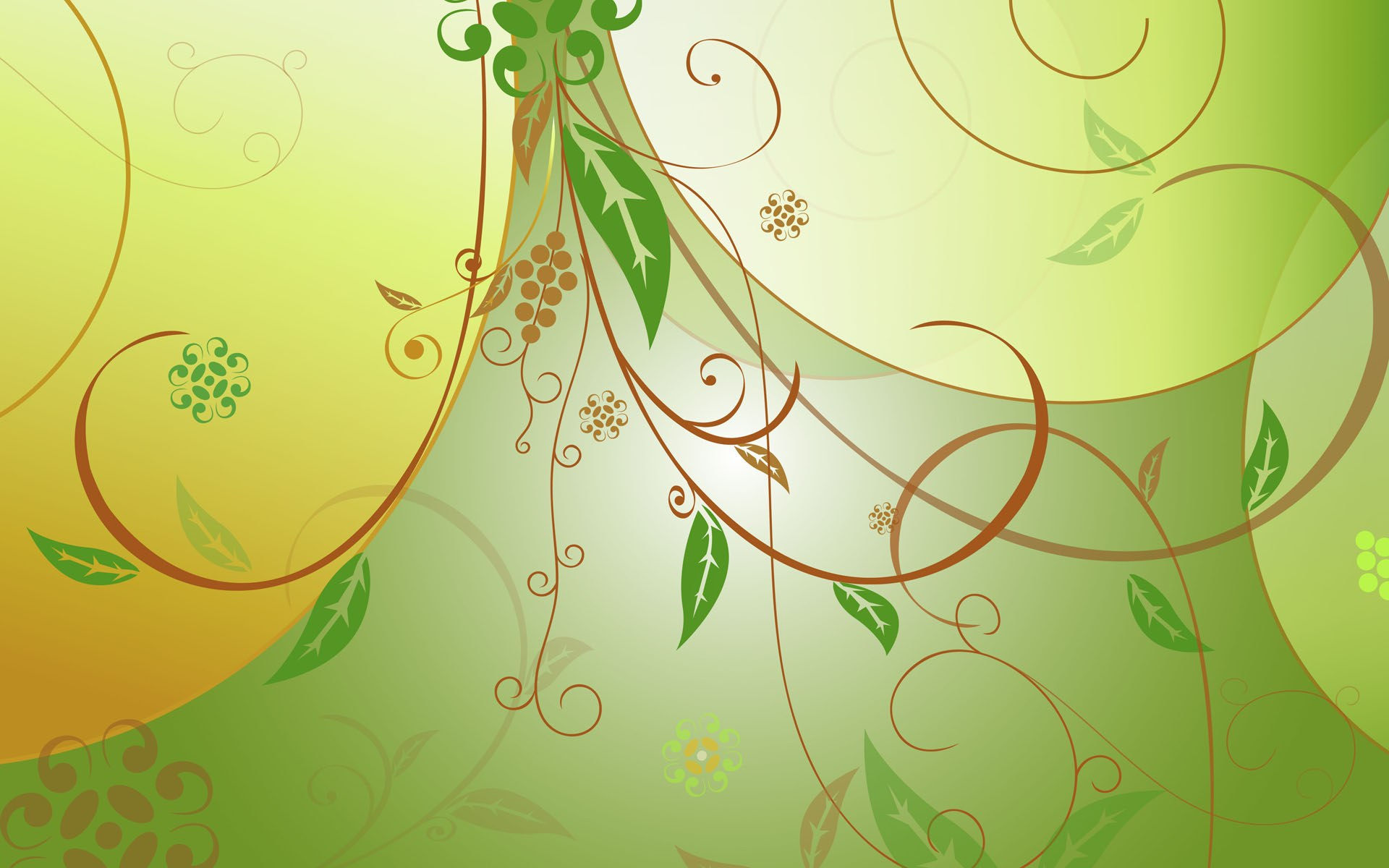

Grade 12 Biology - Unit One

Carbohydrates
Carbs are among the most common organic materials on Earth. They are used by organisms for;
1) Sources of Energy
2) Building materials
3) As cell surface markers for cell-to-cell communication.
Carbohydrates contain carbon, hydrogen, and oxygen in a 1:2:1 ratio. The chemical formula for carbs is (CH2O)n. n represents the number of carbon atoms.
Carbohydrates can be classified into THREE groups;
1) Monosaccharides
2) Oligosaccharides (dissaccharides)
3) Polysaccharides
*The term saccharide and the suffix -ose refer to sugars. Ex. A sugar with five carbons is called a pentose, one with six carbons is a hexose.
Polysaccharides
Polysaccharides are monosaccharide polymers that are made up of several hundred to several thousand monosaccharide subunits held together by glycosidic linkages.
- Some polysaccharides are straight chains, and some are branched.
The two important functions that polysaccharides serve in living cells are;
1) Energy storage. Ex. Starch and glycogen
2) Structural support. Ex. Cellulose and chitin
- Plants store the sun's energy in the form of glucose and other carbs through photosynthesis. When plants produce more glucose than needed, these glucose molecules bond together and form starch, the main energy storage molecule in plants. Starch is a mixture of two different polysaccharides; amylose and amylopectin.
- Amylose is a straight chain a-glucose polymer with 1-4 glycosidic linkages, and amylopectin is a branched-chain a-glucose polymer with 1-4 linkages in the main chain and 1-6 linkages at the branch points.
Monosaccharides
Monosaccharides are the simplest carbohydrates. This class of carbs contains a single chain of carbon atoms which hydroxyl groups are attached. They can be distinguished by the carbonyl group they posess, aldehyde or ketone.
- The two simplest monosaccharides are dihydroxyacetone (a triose with a ketone group) and glyceraldehyde (a triose with an aldehyde group).
- Two common pentose sugars are ribose (a component of RNA) and ribulose (used in photosynthesis). Three common hexoses are glucose (source of energy for ALL cells), galactose (milk sugar), and fructose (fruit sugar).
- The spatial arrangement of the atoms of simple sugars are another distinguishing feature. These include isomers such as glucose, galactose, and fructose, which all have the chemical formula C6H12O6.
Isomers - Molecules with the same chemical formula but with a different arrangement of atoms. They possess different shapes and different physical and chemical properties.
- Monosaccharides with five or more carbons are linear molecules in the dry state, but form ring structures when dissolved in water.
- When a glucose molecules forms a six-membered ring, there is a 50% chance that the hydroxyl group at carbon one will end up below the plane of the ring. When this happens, it forms a molecule called α-glucose. If the hydroxyl group ends up above the plane of the ring, β-glucose is formed.

Oligosaccharides
Oligosaccharides are sugars containing two or three simple sugars attached to one another by covalent bonds called glycosidic linkages.
Glycosidic linkages - Covalent bonds that hold monosaccharides together by condensation reactions where the H atom comes from a hydroxyl group on one sugar, and the -OH group comes from a hydroxyl group of the other sugar.
- Examples of important dissacharides are;
1) Maltose - which is composed of two α-glucose molecules held together by a 1-4 glycosidic linkage. This is found in grains which is used in the production of beer.
2) Sucrose - which is composed of α-glucose and α-fructose with α 1-2 glycosidic linkage between them. This is table sugar.
3) Lactose - Composed of α-glucose and α-galactose. Lactose is normally found in milk.

Maltose
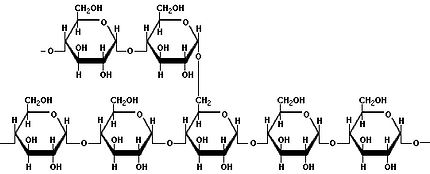

Amylopectin
Amylose
Humans and other plant eaters use the plant's stockpile of energy (starch) as a source of food. Animals can hydrolyze amylose and amylopectin into individual glucose molecules and extract energy from them. However, the human digestive system is unable to break down the B-glucose subunits in cellulose, unlike certain animals like cows, sheep and rabbits. Although humans are unable to use cellulose as a source of energy, it is a healthy part of a well-balanced diet. Cellulose fibres, called roughage, pass through our digestive system and gently scrape the walls of our large intestine, which stimulates cells to secrete mucus and aids in the elimination of solid waste.
Cellulose is the primary structural polysaccharide in all plants, and is a major component in cell walls. It is a straight chain polymer of B-glucose that is held together by 1-4 glycosidic linkages. The straight shape of these molecules allow the hydroxyl groups of parallel molecules to form many hydrogen bonds and produce tight bundles called microfibrils.
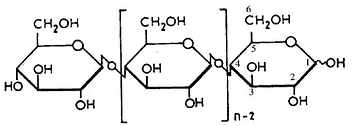
A cellulose-like polymer exists in the hard exoskeleton of insects, crustaceans, and the cell wall of many fungi. This polymer is known as chitin. Chitin is the second most abundant organic material found on Earth. It is a glucose molecule to which there is a nitrogen-containing group attached at the second carbon position. Chitin's physical and chemical properties can also be used in medicine, such as biodegradable stitches that decompose when a wound heals.
Lipids are hydrophobic molecules that are composed of carbon, hydrogen, and oxygen. They contain few polar O-H bonds and more nonpolar C-H bonds than carbohydrates and because of this, they are insoluble in water but soluble in other nonpolar substances. Some are long hydrocarbon chains with attached to smaller subunits and some are compact molecules made up of several hundred hydrocarbon rings.
Organisms use lipids for;
1) Storing energy
2) Building membranes and other cell parts
3) Chemical signalling molecules
Lipids can be divided into four families;
1) Fats
2) Phospholipids
3) Steroids
4) Waxes
Lipids

Fats are the most common energy storing molecules in living organisms. Animals convert excess carbohydrates into fat and store it as droplets in the cells of adipose (fat) tissue which can act as thermal insulation. Plants also store energy in the form of fat.
Proteins
Glycerol is a three-carbon alcohol that has a hydroxyl group attached to each carbon. But fatty acids are long hydrocarbon chains containing a single carboxyl group at one end.
Fats
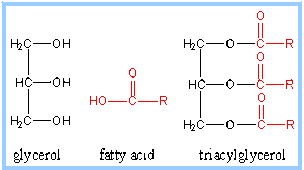
The most common fats in plants and animals are the triacylglycerols which contain three fatty acids attached to a molecule of glycerol.
Unsaturated fatty acids have only single bonds between carbon atoms, whereas unsaturated fatty acids have double bonds between carbons. Saturated fatty acids are saturated because they contain the maximum number of hydrogen bonds, unlike unsaturated fatty acids, where there are fewer than the maximum number of hyrogens possible. Because of this, unsaturated fatty acids have a "kink" in the chain and this causes the reduction of Van Der Waal attractions along their lengths with other unsaturated fatty acids. This is why oil is liquid at room temperature. Saturated fatty acids, however, have no kinks and have straight, hydrocarbon chains, where there are more Van Der Waal attractions along their lengths. This is why animal fats, such as butter and lard, are solids at room temperature. There is an exception to this and this is caused by an industrial process called hydrogenation which adds hydrogen atoms to the double bonds in unsaturated fatty acids, like corn oil or canola oil, and causes them to be solids, such as margarine.
Another class of lipids that are in the cell membrane are sterols, which are compact hydrophobic molecules containing four fused hydrocarbon rings and several different functional groups. Some examples of sterols are cholesterol and sex hormones (testosterone, estrogens, and progesterone).
When glycerol reacts with fatty acids, a hydroxyl group of glycerol and the carboxyl group of a fatty acid go through a condensation reaction. The resulting bond is called an ester linkage.
Cell membranes normally separate two water compartments, the inside of a cell and the outside. Cell membranes are mostly composed of fats called phospholipids. When these phospholipids are added to water, their hydrophilic heads face away from each other and dissolve in the water and their hydrophobic tails face each other away from water molecules. As a result of this, spheres called micelles are formed. Water and other polar and ionic materials cannot pass through the phopholipid bilayer due to the fact that it has a highly nonpolar centre. Proteins and hydrophilic pore form channels where charged materials can pass through the cell membrane.
Waxes are lipids that contain long-chain fatty acids linked to alcohols or carbon rings. They are hydrophobic with a firm, pliable consistency, and this makes them useful for waterproof coatings on plants and certain animal parts. For example, birds secrete a waxy material that helps keep their feather dry and bees produce beeswax to construct honeycombs.
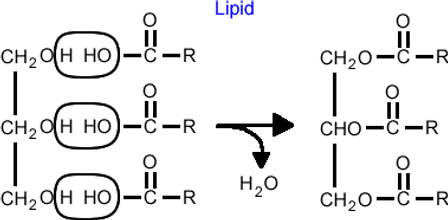
Ester Linkage
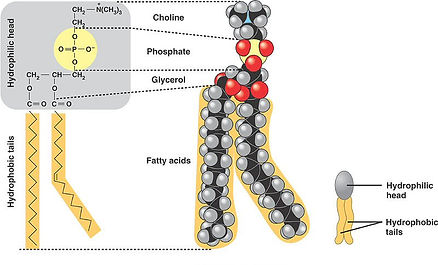
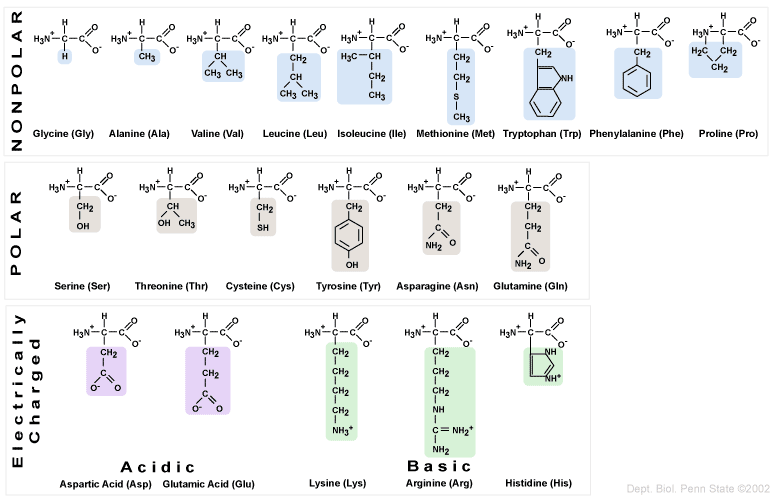
Proteins are the most diverse and most important molecules in living organisms. The genetic information in DNA codes is specifically for the production of proteins and nothing else. They are involved in almost everything that cells do and are used as structural building blocks and as functional molecules.
Enzymes are proteins that act as biological catalysts, which makes chemical reactions occur quicker in order to sustain life.
Proteins are amino acid polymers that are folded into specific three-dimensional shapes. A protein's structure determines its function. Amino acids are organic molecules that contain a central carbon atom attached to an amino group, a carboxyl group, and an R group, which can be any of the 20 different amino acids. Amino acids are amphiprotic, which means that they can possess both acidic (carboxyl) and basic (amino) functional groups. When amino acids are dissolved in water, the carboxyl group donates an H+ ion to the amino group, causing the carboxyl group to be negatively charged and the amino group to be positively charged. Acidic amino acids have a carboxyl group on their side chains, whereas basic amino acids contain amino groups on their side chains.
Some example of proteins are;
- Immunoglobins, which protect animals from foreign microbes and cancer cells.
- Hemoglobin, which shuttles oxygen from place to place in mammals.
- Protein carriers, which help move sucrose through phloem tissue in plants.
- Keratin, the most common protein in vertebrates, is found in hair and fingernails
- Fibrin, a protein that helps a blood clot.
- Collagen, which forms the protein component of bones, skin, ligaments and tendons.
Examples:
- Glycine is the simplest amino acid with a single hydrogen atom in its R group.
- Proline is the only amino acid whose side chain forms a covalent bond with its own amino group.
Proteins have one or more amino acid polymers (polypeptides) that have twisted and coiled into a specific shape.
Conformation - The three-dimensional shape of a protein determined by the sequence of amino acids it contains.
There are multiple copies of the 20 amino acids dissolved in a cell's cytoplasm and these amino acids are constructed by the cell from simpler compounds or obtained from the diet. However, there are 8 essential amino acids that MUST be obtained from the diet. These are;
1) Tryptophan
2) Methionine
3) Valine
4) Threonine
5) Phenylalanine
6) Leucine
7) Isoleucine
8) Lysine
Peptide bonds - The amide linkage that holds amino acids together in polypeptides.
Peptide bonds are formed by a condensation reaction between the amino group of one amino acid, and the carboxyl group of an adjacent amino acid.
Globular proteins are composed of one or more polypeptide chains that take on a rounded, spherical shape. Ex. Many enzymes and other functional proteins are globular.
There are four levels of structure in globular proteins;
1) Primary
2) Secondary
3) Tertiary
4) Quaternary
- Primary, secondary and tertiary apply to individual polypeptide chains of a protein and Quaternary applies to the interactions that occur between polypeptide strands in proteins composed of two or more polypeptides.
All copies of a polypeptide will have the same sequence of amino acids because of a gene in a DNA molecule. These sequences can range in length from a few amino acids to more than a thousand! A polypeptide chain will always have an amino group (amino terminus) at one end and a carboxyl group (carboxyl terminus) at the other end. Many structural proteins are linear in shape and are arranged to form strands or sheets. This is where globular proteins come in.

Primary structure is when a unique sequence of amino acids are in a polypeptide chain. An amino acid subunit is referred to as a residue. Primary structure is determined by the nucleotide sequence in a gene in DNA. This nucleotide governs the order in which indiviudal amino acids link together to form a polypeptide chain. The final conformation of a protein is very sensitive to its primary structure, because that's where it all begins. An example of this may be sickle cell anemia, where just ONE single amino acid substitution changes the conformation of a polypeptide in hemoglobin, and this causes red blood cells to be deformed.
As the chain grows from its primary structure, it begins to coil and fold at various locations. These coils and folds are referred to as the polypeptide's secondary structure. These coils and folds are created when a hydrogen bond forms between the electronegative oxygen of the carboxyl group C=O of one peptide bond and the electropositive hydrogen of an amino group N-H, which is 4 peptide bonds away. When this is repeated over a certain length of the chain, the polypeptide forms into a helix. An example of a helical protein may be a-kertin, found in hair. Secondary polypeptides may also form B-pleated sheets, which occurs when two parts of the polypeptide chain are parallel to one another. An example of this may be lyzosome, the natural disinfectant in saliva, sweat, and tears.
Strong forces of attraction and repulsion undergo between the polypeptide and its environment. This forces it to go additional folding into a tertiary structure. Tertiary structure is stabilized by hydrogen bonds between certain polar side chains, ionic bonds between oppositely charged side chains and van der Waal forces between nonpolar R groups. Another strong stabilizer that helps hold tertiary structure together is a disulfide bridge, which is formed when the sulfur-containing R groups of two cysteine residues are brought close to one another and form a covalent bond.
When two or more polypeptide subunits come together to form a functional protein, they are said to be in quaternary structure. Some examples of polypeptides in this structure are collagen (found in skin, bones, tendons, and ligaments), keratin (found in hair and nails), and hemoglobin (transports oxygen in red blood cells)
A polypeptide's final shape is determined by its primary structure, BUT chemical and physical factors may effect the final shape of the molecule. Take for example, if heat is applied to hair when it is straightened or curled, it denatures the proteins in the hair. A denatured protein cannot carry out its biological function and various chemicals and heat may disrupt the hydrogen bonds, ionic bonds, disulfide bridges, and hydrophobic interactions that hold a polypeptide in tertiary structure. However, smaller proteins are able to refold themselves into tertiary structure if the denaturing agent is removed, but larger proteins are unable to refold spontaneously like this. In order for larger globular proteins to refold, chaperone proteins aid the growing polypeptide to fold into tertiary structure.